Here are some of my thoughts on education and mentoring together with other reference materials I like. Please do not quote or cite without consulting Ozgur Keles.
2023 Update: After long hours of edits, updates, tests, and analyses, we have our results published. You can download our current VLE from GitHub. Let me know if you have any questions.
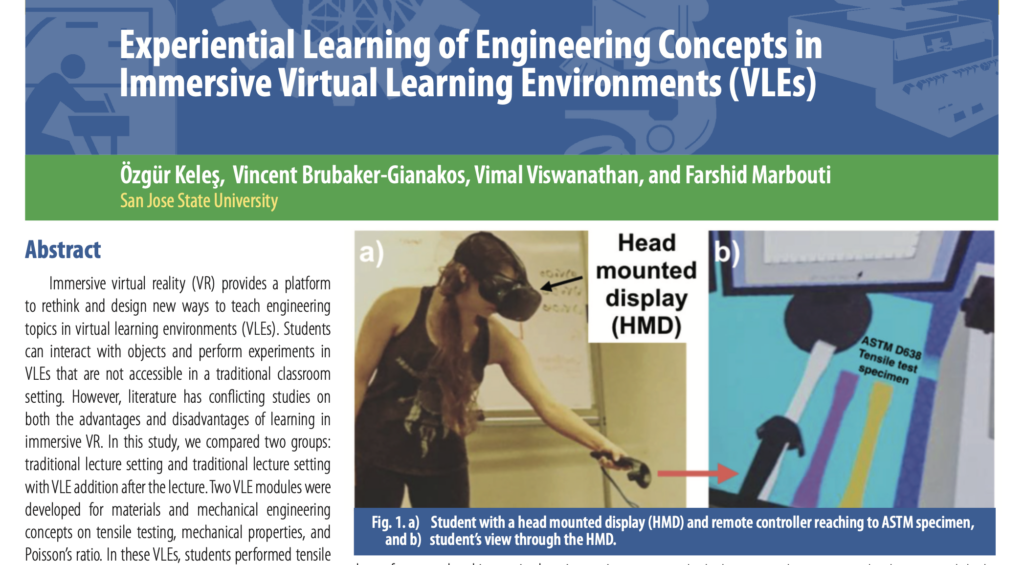
Abstract: Immersive virtual reality (VR) provides a platform to rethink and design new ways to teach engineering topics in virtual learning environments (VLEs). Students can interact with objects and perform experiments in VLEs that are not accessible in a traditional classroom setting. However, literature has conflicting studies on both the advantages and disadvantages of learning in immersive VR. In this study, we compared two groups: traditional lecture setting and traditional lecture setting with VLE addition after the lecture. Two VLE modules were developed for materials and mechanical engineering concepts on tensile testing, mechanical properties, and Poisson’s ratio. In these VLEs, students performed tensile tests on real-size samples and explored material behavior with different properties using hand controllers. In-VLE questions on mechanical properties were asked after virtual tensile testing. Student learning was improved for only tensile testing concepts. Despite the significant hand-controlled interaction with the materials in the Poisson’s ratio VLE, student learning was not improved with the addition of VLE after the traditional lecture. These results show that the VLEs need to be carefully designed following the main affordances of immersive VR and multimedia learning theories to enhance student learning.
Pre-2020:
I aim to improve student learning by virtual learning environments (VLEs). We design and develop various virtual reality (VR) environments related to mechanics of materials and nanocomposite structures. VR is a platform for students to experience a VLE that is designed by educators to facilitate learning. These VLEs have the potential to revolutionize engineering education by providing heretofore unexplored immersive learning environments.
My engineering education research motivation is based on four education gaps. 1) Global competitiveness: In 2013, U.S. universities granted 88,000 engineering degrees, 4.7 % of all bachelor’s degrees. Meanwhile, China in 2013 and India in 2011 produced 965,000 (32 % of all degrees) and 550,000 (10 % of all degrees) engineers, respectively [a]. 2) Equity and diversity: Women earned 19 % of the engineering degrees in U.S. in 2012 (about the same as in 2007, 18.5 %), and members of racial and ethnic minorities are still underrepresented in engineering fields [a]. 3) Increasing skill gap: 700,000 manufacturing jobs were unfilled due to lack of skilled workforce in 2015 [b] compared to 600,000 in 2011 [c]. 4) STEM professionals gap: We will need 1 million more STEM professionals by 2022 due to economic projections [d]. Inclusion of VLEs in engineering classroom can help address these four gaps and attract more students to engineering disciplines, because all students (including females/minorities) like the new educational technology. Students spend more time in VLEs and learn more; thus, help retention of struggling students in engineering. Low interest manufacturing fields can be taught in an exiting way via VLEs and generate the required workforce.
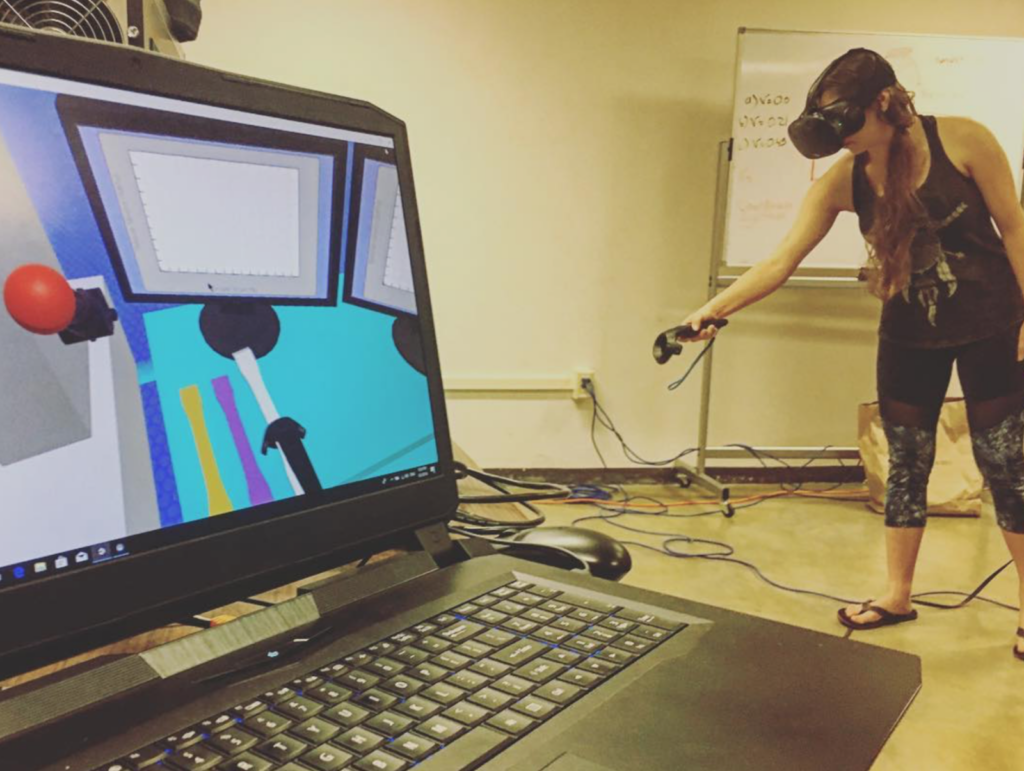
Virtual interaction with materials to observe mechanical properties. A closer look to Poisson’s ratio.
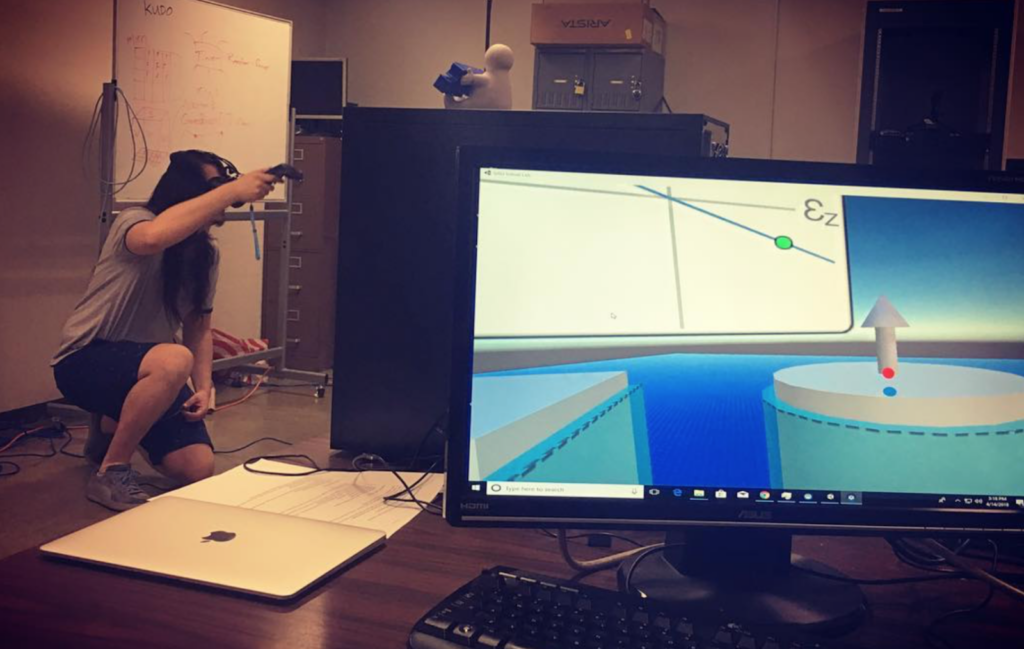
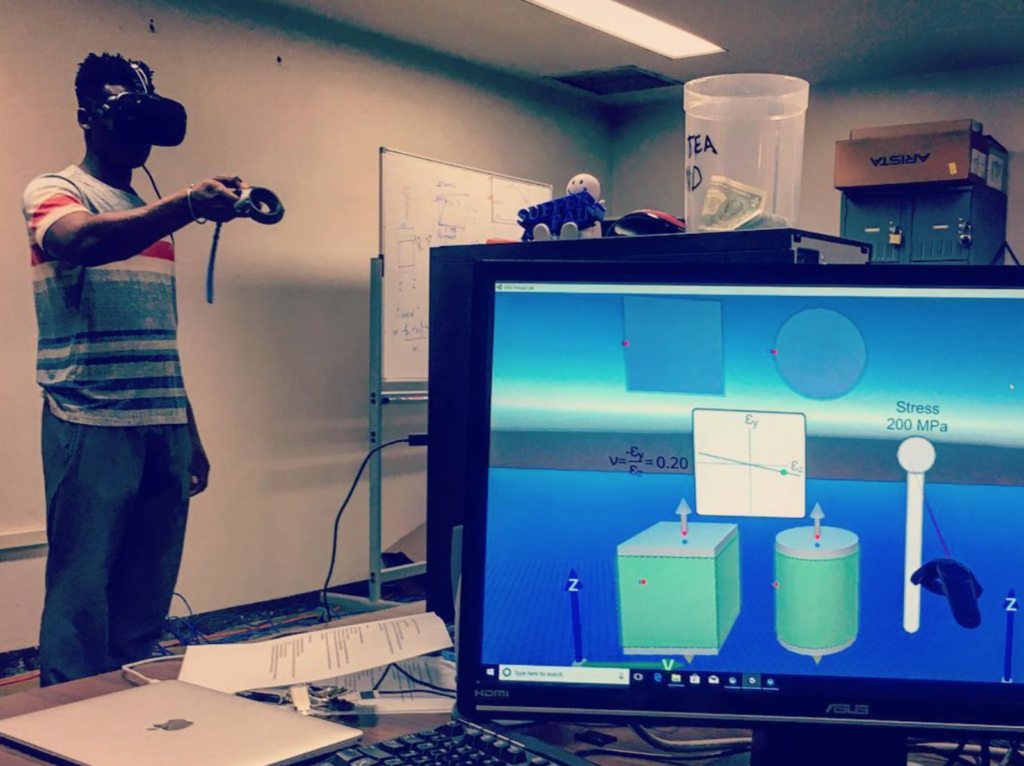
[a] N. S. Board, Science and Engineering Indicators 2016, National Science Foundation (NSB- 2016-1), Arlington, VA, 2016.
[b] C. Gif, J. McNelly, B. Dollar, G. Carrick, M. Drew, B. Gangula, The skills gap in U.S. manufacturing 2015 and beyond, Technical Report, Deloitte and The Manufacturing Institute, 2015.
[c] T. Morrison, E. S. DeRocco, B. Maciejewski, J. McNelly, C. Gif, G. Carrick, Boiling point? The skills gap in U.S. manufacturing, Technical Report, Deloitte and The Manufacturing Institute, 2011.
[d] P. C. of Advisors on Science, Technology, Engage to Excel: Producing One Million Additional College Graduates with Degrees in Science, Technology, Engineering, and Mathematics, Technical Report, 2012.
Our work in 2017, interviewed by Wareable.com, click to read the whole story:
“When you think of universities exploring new technologies, you tend to think of big research projects that take years, with students and faculties pulling together in one direction. Then there’s San Jose State University’s VR Lab, which takes the opposite approach.
New paper: Peggy Boylan-Ashraf, Ozgur Keles, Steve Freeman, Mack Shelley, Robert Calfee, Can Students Flourish in Engineering Classrooms?, Journal of STEM Education: Innovations and Research, Accepted.
Some of our pre-2017 work on learning:
Our education goal is to cultivate creative engineers using photography and virtual reality (VR) in support of the Science, Technology, Engineering, Arts, and Mathematics (STEAM) movement. The National Academies’ “Rising Above the Gathering Storm” committee indicated that science and engineering innovation is the key for the economic development and job creation [1]. Innovation is strongly correlated to creativity especially at individual level [2, 3]. Therefore, creativity is a must for the next generation engineers to provide innovative solutions for global problems and local problems [4–6]. Studies indicated that addition of arts into STEM fields (STEAM) could increase creativity; thus, a STEAM approach has the potential to cultivate creative engineers who can innovate [7–10]. We are expanding our visual-heavy approach in teaching materials engineering using photography and VR.
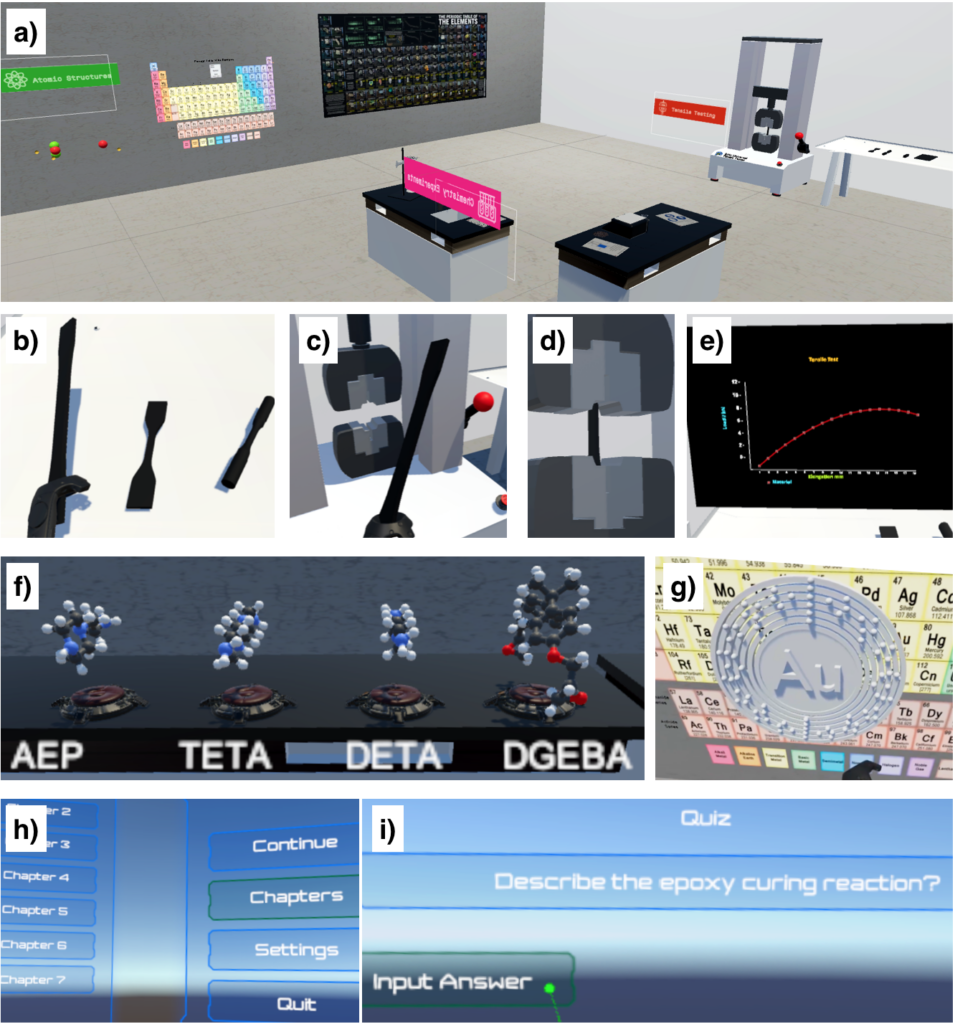
Figure: VR environment showing a) overview of one of our virtual laboratories, b) ASTM D638 Type I and IV tensile specimens, c-d) students can hold and put the specimen to the tensile grips, and e) stress-strain diagram that is generated during the virtual tensile test, f) common epoxy curing agents and resin molecules, g) electronic structure of gold, h) introduction page to select chapters, and i) example question to be answered in the learning module.
Our educational motivation is based on four education gaps. 1) Global competitiveness: In 2013, U.S. universities granted 88,000 engineering degrees–4.7 % of all bachelor’s degrees. Meanwhile, China in 2013 and India in 2011 produced 965,000 (32 % of all degrees) and 550,000 (10 % of all degrees) engineers, respectively [11]. 2) Equity and diversity: Women earned 19 % of the engineering degrees in U.S. in 2012 (about the same as in 2007, 18.5 %), and members of racial and ethnic minorities are still underrepresented in engineering fields [11]. 3) Increasing skill gap: 700,000 manufacturing jobs are unfilled due to lack of skilled workforce in 2015 [12] compared to 600,000 in 2011 [13]. 4) STEM professionals gap: President’s Council of Advisors on Science and Technology said we will need 1 million more STEM professionals by 2022 due to economic projections [14]. San Jose State University is an ideal place to address these gaps and introduce a STEAM approach to under(graduate) education. SJSU is at the heart of the Silicon Valley, which constantly seeks innovative engineers.
We will use VR to facilitate learning, improve creativity, and increase student involvement [15–21]. Most people at or above college age are visual learners [22]: e.g., 82 % of engineering students are visual learners [22–25]. Meanwhile, most college education is oral, i.e., lecturing and/or presentation of words or mathematical symbols. We aim to enhance visual communication in materials engineering education via photography and VR and so address 1) global competitiveness by increasing the number of creative/innovative engineers, 2) equity and diversity by graduating more minorities/women at SJSU, 3) reduce the skill gap by providing an open-source learning platform for materials science and engineering, and 4) reduce the STEM professionals gap by attracting and graduating more engineers. In addition, people with disabilities of physical functioning or mobility, i.e., students who cannot physically perform the experiments, will benefit immersive laboratories and virtual experiments. Therefore, VR learning environment can open up a new way of knowledge transfer to physically disabled.
Faculty and instructors have also used VR to teach/train learners about additive manufacturing, manufacturing, machining, assembly, and product development [36–38, 17]. We are developing VR materials laboratory environments, where students can perform tensile tests, generate molecules, visualize electronic structure, and visualize Brownian motion (Figure above). We are extending our VR-lab to include fracture toughness tests and nanoindentation proposed in this proposal and include visualization of GQD/GNP-epoxy hierarchical composites, thus, extend our open-source platform aimed at democratizing engineering education using VR. We use the Unity game engine to generate VR environments. Unity is a cross-platform tool that allows the developed content to be played in VR, augmented reality, browsers, or consoles with minimal effort. Our goal is to use student mobile phones for VR education via cheap Google Cardboard headsets. The VR modules will include videos that show how to perform the tensile tests, toughness tests, nanoindentation, and ask students to perform these virtual experiments. We will test the student learning by asking questions in the VR modules. We are testing the modules on a set of 10 students regularly during the design process. In 2018, one section of our introductory materials class will be assigned to the VR-homework. Students will be able to access VR-equipment in the SJSU’s VR laboratory or use their mobile phones.
We will test the creativity of the students using Wallach–Kogan creativity test, which can be given in class and asks questions such as “Name all the round things you can think of.” [34, 35]. The scores are based on originality, number of responses, flexibility (number of different categories), and elaboration. We will collect creativity results from the introductory materials class at the beginning and end of the semester from two sections with ~160 students. Only one of the sections will use VR in class; thus, we will observe the effects of visual-heavy teaching approaches on creativity.
References
[1] N. A. of Sciences, N. A. of Engineering, I. of Medicine, Rising Above the Gathering Storm, Revisited: Rapidly Approaching Category 5, The National Academies Press, 2010.
[2] H. Sarooghi, D. Libaers, A. Burkemper, Examining the relationship between creativity and innovation: A meta-analysis of organizational, cultural, and environmental factors, Journal of Business Venturing 30 (2015) 714–731.
[3] I. Badran, Enhancing creativity and innovation in engineering education, European Journal of Engineering Education 32 (2007) 573–585.
[4] R. M. Felder, Creativity in engineering education, Chemical Engineering Education 22 (1988) 120–125.
[5] R. M. Felder, On creating creative engineers, Engineering education 77 (1987) 222–227.
[6] K. F. Lallement, D. J. Fallon, Finding parallels: Advancing creativity and innovation in engineering through the visual arts (2015).
[7] A. M. Connor, S. Karmokar, C. Whittington, From stem to steam: strategies for enhancing engineering & technology education (2015).
[8] Y. Kim, N. Park, The effect of steam education on elementary school student’s creativity improvement, in: Computer Applications for Security, Control and System Engineering, Springer, 2012, pp. 115–121.
[9] G. A. Boy, From stem to steam: toward a human-centred education, creativity & learning thinking, in: Proceedings of the 31st European Conference on Cognitive Ergonomics, ACM, p. 3.
[10] M. E. Madden, M. Baxter, H. Beauchamp, K. Bouchard, D. Habermas, M. Huff, B. Ladd, J. Pearon, G. Plague, Rethinking stem education: An interdisciplinary steam curriculum, Procedia Computer Science 20 (2013) 541–546.
[11] N. S. Board, Science and Engineering Indicators 2016, National Science Foundation (NSB-2016-1), Arlington, VA, 2016.
[12] C. Gif, J. McNelly, B. Dollar, G. Carrick, M. Drew, B. Gangula, The skills gap in U.S. manufacturing 2015 and beyond, Technical Report, Deloitte and The Manufacturing Institute, 2015.
[13] T. Morrison, E. S. DeRocco, B. Maciejewski, J. McNelly, C. Gif, G. Carrick, Boiling point? The skills gap in U.S. manufacturing, Technical Report, Deloitte and The Manufacturing Institute, 2011.
[14] P. C. of Advisors on Science, Technology, Engage to Excel: Producing One Million Additional College Graduates with Degrees in Science, Technology, Engineering, and Mathematics, Technical Report, 2012.
[15] C. Haskell, Understanding quest-based learning, White paper. Boise State University (2013).
[16] T. M. Connolly, E. A. Boyle, E. MacArthur, T. Hainey, J. M. Boyle, A systematic literature review of empirical evidence on computer games and serious games, Computers & Education 59 (2012) 661– 686.
[17] F. M. Dinis, A. S. Guimarães, B. R. Carvalho, J. P. P. Martins, Virtual and augmented reality game- based applications to civil engineering education, in: Global Engineering Education Conference, IEEE, pp. 1683–1688.
[18] B. D. Coller, D. J. Shernoff, Video game-based education in mechanical engineering: A look at student engagement, International Journal of Engineering Education 25 (2009) 308.
[19] B. D. Coller, M. J. Scott, Effectiveness of using a video game to teach a course in mechanical engineering, Computers & Education 53 (2009) 900–912.
[20] G. Barata, S. Gama, J. Jorge, D. Gonçalves, Engaging engineering students with gamification, in: Games and Virtual Worlds for Serious Applications (VS-GAMES), 2013 5th International Conference on, IEEE, pp. 1–8.
[21] G. Barata, S. Gama, J. Jorge, D. Gonçalves, So fun it hurts–gamifying an engineering course, in: International Conference on Augmented Cognition, Springer, pp. 639–648.[22] R. M. Felder, L. K. Silverman, Learning and teaching styles in engineering education, Engineering education 78 (1988) 674–681.
[23] A. Kolmos, J. E. Holgaard, Learning styles of science and engineering students in problem and project based education, in: SEFI 36th Annual Conference.
[24] R. M. Felder, R. Brent, Understanding student differences, Journal of engineering education 94 (2005) 57–72.
[25] R. M. Felder, J. Spurlin, Applications, reliability and validity of the index of learning styles, International journal of engineering education 21 (2005) 103–112.
[26] M. Munakata, A. Vaidya, Encouraging creativity in mathematics and science through photography, Teaching Mathematics and its Applications (2012) hrr022.
[27] J. E. Machin, Show, don’t tell: Using photographic “snapsignments” to advance and assess creative problem solving, Marketing Education Review 26 (2016) 8–13.
[28] Ö. Keleṣ, Students blogging about materials, www.ozgurkeles.com/2017/06/students-blogging-on- materials.html, 2017.
[29] J. P. Guilford, R. Hoepfner, The analysis of intelligence, McGraw-Hill Companies, 1971.
[30] C. R. Østergaard, B. Timmermans, K. Kristinsson, Does a different view create something new? the effect of employee diversity on innovation, Research Policy 40 (2011) 500–509.
[31] N. Duarte, Slide: ology: The art and science of creating great presentations, O’Reilly Media Sebastopol, CA, 2008.
[32] F. Frankel, A. H. DePace, Visual strategies: a practical guide to graphics for scientists & engineers, Yale University Press, 2012.
[33] E. R. Tufte, P. Graves-Morris, The visual display of quantitative information, volume 2, Graphics press Cheshire, CT, 1983.
[34] M. A. Wallach, N. Kogan, Modes of thinking in young children. (1965).
[35] L. Zeng, R. W. Proctor, G. Salvendy, Can traditional divergent thinking tests be trusted in measuring and predicting real-world creativity?, Creativity Research Journal 23 (2011) 24–37.
[36] A. Renner, J. Holub, S. Sridhar, G. Evans, E. Winer, A virtual reality application for additive manufac- turing process training, in: ASME 2015 International Design Engineering Technical Conferences and Computers and Information in Engineering Conference, American Society of Mechanical Engineers.
[37] A. A. Kadir, X. Xu, E. Hämmerle, Virtual machine tools and virtual machining—a technological review, Robotics and computer-integrated manufacturing 27 (2011) 494–508.
[38] L. Schina, M. Lazoi, R. Lombardo, A. Corallo, Virtual reality for product development in manufacturing industries, in: International Conference on Augmented Reality 2016, Virtual Reality and Computer Graphics, Springer, pp. 198–207.
On education, 2016:
Can we better facilitate learning in engineering education using arts? The figure given below shows sets of images–all taken by me–that are related to fracture and microstructure. First set of images (a) to (d) includes (a) rose, (b) crack front in a rose petal, (c) fracture surface of the petal, and (d) surface morphology of the petal. The second set of images (e) to (i) shows (e) surface of a 3D printed ABS containing a circular hole, (f) internal microstructure just below the top surface of 3D printed ABS in (e), (g) fracture surface near the hole, and (i) higher magnification image of (g). These two sets of images are both related to fracture phenomenon and microstructure. Based on my experience, showing the first set of images and then showing the second set to explain the fracture in additively manufactured materials results in a higher level discussion on the subject and more questions from the students. I plan to investigate the effects of using arts in engineering education in a more systematic way to quantify the possible benefits in learning.
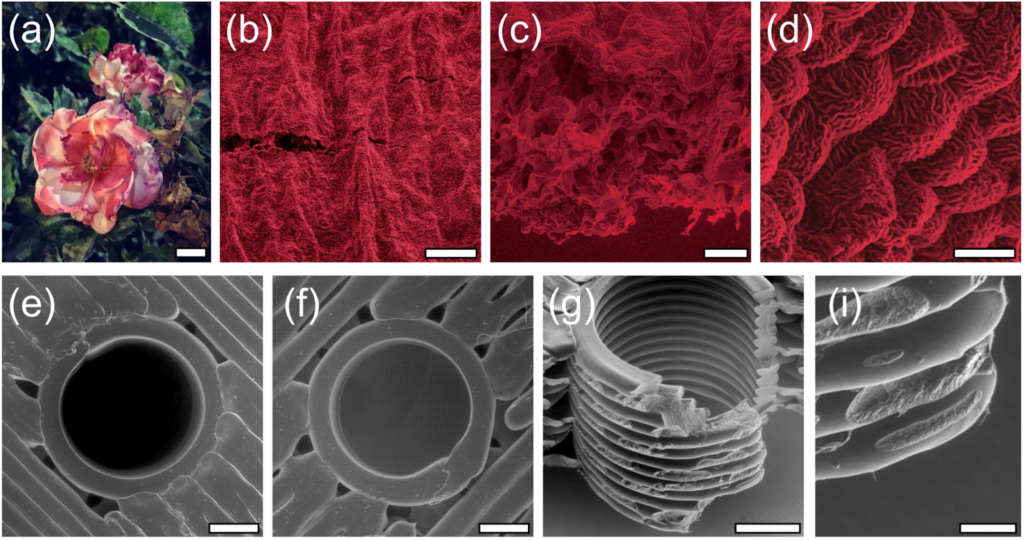
Figure: Multi-scale arts consisting of a) life size artistic images and high magnification SEM images that shows b) crack bridging, c) fracture surface, and d) intricate surface morphology of a rose petal. Fused deposition modeled ABS e) top surface raster, f) internal raster with thicker roads, g) fracture surface around the hole, and i) fracture surface at the road level. Scale bars represent a)10 mm, b) and c) 50 μm, d) 10 μm, e), f), and g) 1mm, and i) 100 μm.
Pre 2012:
On public education (Thanks to Jessica Mehr for the edits):
The use of technology in public education is still in its infancy, severely limiting hands-on learning opportunities. At the same time, the American education system promotes a generalized curriculum drastically longer than most Western nations; students often do not choose their focus field until their sophomore or junior year of college, and such decisions are often based on practicalities, not genuine passion.
If we could help students to discover their educational passions earlier in life—preferably between the ages of 5-12—and then facilitate the development of these passions via technology, we could potentially revolutionize the educational system.
Technology and education should be integrated in two major ways:
1) To identify student passions/interests at a very young age.
2) To provide a platform for facilitating personal, creative, and intellectual success.
1) Identifying Student Interests
Identifying a child’s educational passions at an early age could allow for a level of focused, thorough study that is relatively unheard of in America’s current educational environment. Through early detection, we could develop a lifelong learning path for our students. But how does one predict the lifelong educational passions of a five-year old? Innovative technologies could potentially identify innate interests that the child is not conscious of or able to communicate.
Dynamic systems could relate students’ physical movements to their interests in particular environments. For example, if students are given the chance to spend an afternoon in a science museum, tracking devices could relate the time spent at different sections to their interests. Thus, a student who spends more time in a botanical garden could be associated with a higher interest in biology. Alternatively, a student who spends more time in the space section might be regarded as being more technology-oriented. Google glass will be a flexible tool to understand how people learn and improve education, especially with the addition of an eye tracking feature.
Likewise, static systems could reveal interests by using electroencephalography (EEG), pressure and movement sensors, and cameras in schools and museums. Obviously, implementing these strategies would require pilot programs in which parents/guardians provide express permission for students to be monitored from a distance. In such an environment, students’ brain activity could be measured via EEG while students are shown pictures on different topics such as arts, literature, mathematics, and science. If a student shows a higher brain activity when viewing the arts, further analysis could be conducted on subtopics such as photography, collage, dance, theatre, and music to help them discover their talents. Unlike the known practice of using EEG once or several times, I am suggesting the use of EEG in regular periods to detect changes in interests and their possible correlation with topics learned or associated activities. The use of EEG could be standardized as equipment such as Emotive becomes cheaper. In addition, electrodermal activity (EDA) can be used to measure skin conductivity through wearable sensors [1]. The change in skin conductivity is related to emotion, cognition, and attention [2]. Hence, a comfortable wrist band sensor [2] can provide a measure for student involvement for specific tasks and activities.
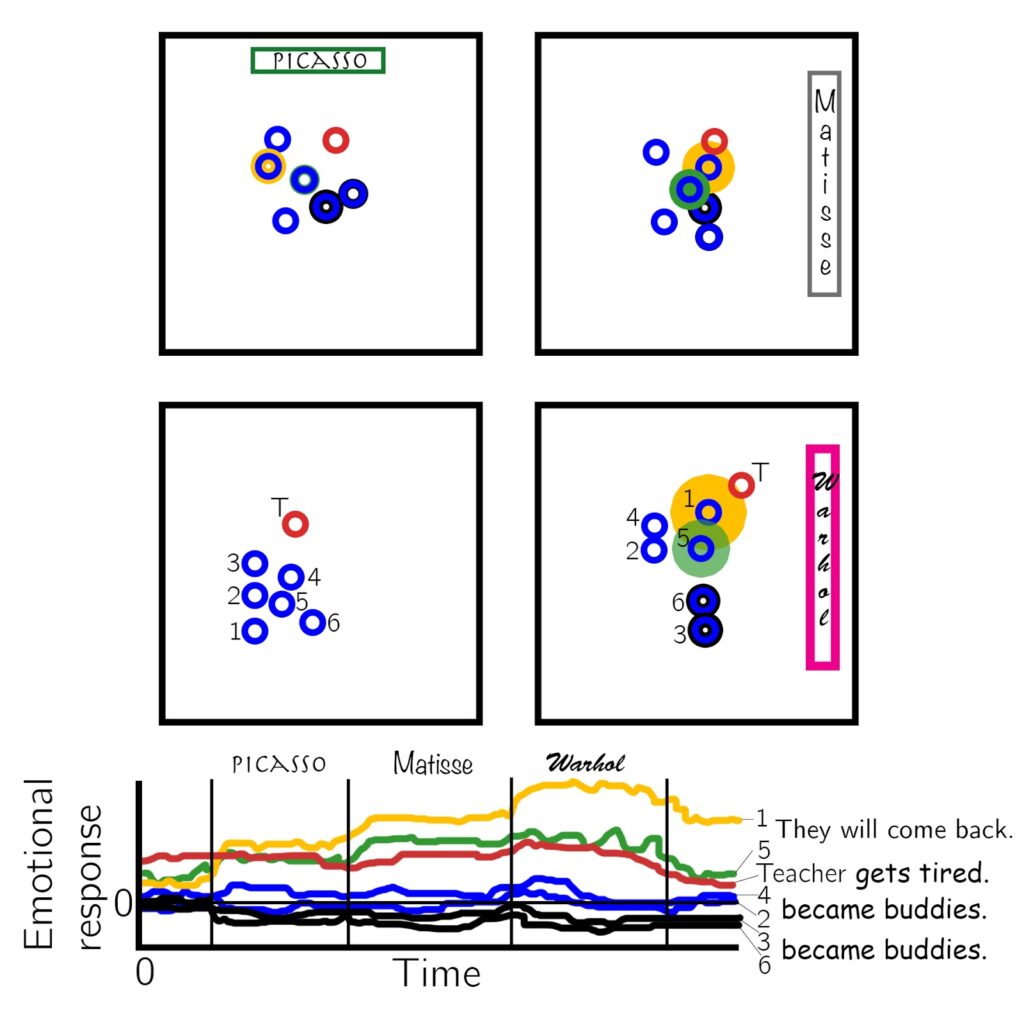
Figure showing an imaginary museum education situation with emotional responses (skin conductivity) of six students (blue circle) and a teacher (red circle). Positions can also be gathered to generate a point cloud for further correlation. Warhol seems to be favored by some of the students.
We could potentially implement pilot “sensor schools” that monitor student behavior through sensors and cameras. By this way, data collected from pressure sensors embedded in chairs and tables could be used to distinguish people that have hard time staying still in the classroom. Different types of learning could also be identified; tactile, visual, and auditory learners could be distinguished early on to provide each child with an optimal learning environment.
Once a student’s innate talents and interests are identified, students can begin to cultivate these skills at a drastically younger age. Rather than forcing students to spend equal time on all subjects, they can be given added challenges in the field that interests them most; by finding his/her passion, students will realize that school does not have to be a collection of onerous tasks, but can be source of great fun and personal reward.
2. To provide a platform for facilitating personal, creative, and intellectual success.
It is well established that hands-on learning is an essential supplement to classroom-based discussion; however, at the elementary-school level, hands-on learning is often added sporadically via activities and field trips, and students are rarely able to integrate these experiences into a long-term learning trajectory.
Technologies, such as Scratch-based web design and 3D printing, could offer young students the ability to take seemingly disparate educational experiences and understand them in the context of a lifelong educational plan.
a. Scratch-based web design
If students began a personal website at an early age, it could serve as a life-long portfolio, one that demonstrates their practical understanding of the information they learned in class. This website would be an open-ended project allowing to students to showcase their accomplishments and self-assess their educational progress. Such a web site might initially consist of a basic biography, interests, hobbies, etc. Students could learn effective collaborative techniques by reviewing each other’s website and providing peer feedback. During such reviews, each website would develop visitor statistics that could be used to teach basic statistics at an early age. In addition, the process of creating and maintaining such a website would improve communication skills, promote creativity, and teach effective design/presentation techniques crucial to any professional or academic field.
An adapted version of the Scratch–a programming language–developed by the Lifelong Kindergarten Group at the MIT Media Lab would be ideal for such an endeavor. Utilizing a tool such as Scratch would allow students to document their hands-on learning accomplishments via a hands-on technological tool. Scratch introduces students to the world of web design at a level that is accessible, encourages creativity, and familiarizes them with basic coding, paving the way for an advanced understanding of coding languages in the future.
b) Scratch-based 3D Printing
In addition to developing a web-based portfolio to which students can add throughout their academic career, 3D printing can be introduced in to public education in order to cultivate student’s creativity and self-expression. I am confident that 3D printing technologies will dominate the future of manufacturing, affecting not just traditional industries such as automotive and household products, but food, fashion, construction, etc. A modified version of Scratch could be used generate 3D shapes and communicate with the 3D printer. Students could use this program to create art pieces, build machine parts, or even design new ways to present meals or chocolates at restaurants. If they have bigger projects to pursue as individuals or groups, they should be encouraged to seek funding for their projects on Kickstarter or similar sources.
Conclusion
If pilot programs utilizing these advanced technologies to identity and cultivate student interests are successful, they could then be applied at the state, national, and even global level. I believe that our inability to solve major world problems (energy, climate, water, food, modern slavery, etc.) is not due to a lack of solutions; it is due to a lack of properly educated and passionate problem solvers. An aggressive and innovate technology-based program provides the best opportunity for nurturing an entire generation of such dedicated intellectuals.
Mentoring the world
The ultimate degree that makes one`s future look the brightest: PhD. Yes, under a lower unemployment rate, those doing their PhDs always publish papers, sometimes write a book, but rarely become famous, and never… Is there anything you imagine that Ph.D.s never do? Not really. So, they also do regular stuff and have their own fallacies like the rest of the society. Their difference lies in their higher academic achievements, which, I believe, are motivated by their mentors.
Let’s take a look at the life of someone doing PhD. It is a good life, at least for the first three years of PhD. Getting a great education is like winning the powerball. You enroll in a Ph.D. program, start working on important projects, and think of making a difference. However, you also sweat over a single topic for a long time, listening to your advisor, feeling unlucky and constrained by the circumstances. This is the opposite of what many would believe lucky people’s lives look like. Richard Wiseman claims that lucky people are those who maximize their chances, rely on their intuition, think they are indeed lucky, and know how to turn bad luck into good luck. Therefore, my question is whether or not Ph.D.s are unlucky people living a good life. And if our answer is yes they are, I believe it is the educators who impose this life on them due to the educational system.
This brings us to the master-apprentice type of education. Many masters (advisors or professors) take for granted the survivor of the fittest approach in science and, therefore, they neglect their main role of being a mentor. Ken Robinson describes mentoring as recognizing, encouraging, facilitating, and stretching. Accordingly, research should be interest-driven and should not be an imposed fixed topic. Professors should help students develop a vision, aim high, and cultivate the habit of hard work. The educational environment should promote sharing and be more tolerant of possible failures. The governing idea should be that we can extend our capabilities and achieve no matter what. Do you think, however, that this is the current practice? I can almost hear you saying “no.”
Even the most prominent professors do a poor job in mentoring the best students of the world. Most of these successful young people do not even get close to realizing their full potential. They use their talents only partially to make money for living. So, if we cannot educate a handful of top-of-the-cream students, how are we supposed to educate others and help them reach their full potentials? The solution is good mentoring. Your mentor can upgrade you to the class of the lucky by providing helpful guidance and motivation. Human brain is capable of handling much more than we imagine. For this reason, we must seek good mentoring that can transform us into independent, self-motivated, and creative individuals.
Primarily because of my co-advisor Dr. Keith J. Bowman, I am interested in engineering education, as well. My short-term goal is to introduce interactive self-education modules, which are based on Mathematica’s computable document format(CDF); please check my demonstration-Weibull distribution fit to computer generated fracture data. In the long run, I will integrate these interactive documents into traditional classroom teaching by using either touch tables (a use is given in the talk by Bang Wong) or students’ touch pads; Eric Schulz talks about his teaching experience in here. I am currently working on a fracture module that will be a part of the Material Science Academy project started by Dr. Arda Çetin. An example application in Turkish showing the elastic shape change can be seen here.
Here are some of engineering education materials on which I will elaborate in the future. The papers and books suggested below provide a good introduction to the subject. I thank H. Onan Demirel for his literature suggestions.
A case study of major curriculum reform effort at Purdue Universtiy carried out by the School of Engineering Education, the first program of its kind in the United States, can be found in:
Judith S. Zawojewski, Heidi Diefes-Dux and Keith J. Bowman (Eds.), Models and Modeling in Engineering Education: Designing Experiences for All Students, Sense Publishers, 2008.
Dr. Frank G. Splitt’s papers from Northwestern University target important questions regarding the future of engineering education and include a wide range of essential readings as references. Example quotations from his papers are given below.
F.G. Splitt, Engineering education reform: A trilogy, The International Engineering Consortium, Chicago, 2003.
“1. Why is the large change such as that implied by ‘engineering education reform’ needed?
2. Why cannot incremental change make the changes needed on an appropriate time scale?
3. Why do we need to recognize leadership and systemic change in engineering education now?”
F.G. Splitt, The Challenge to Change: On Realizing the New Paradigm for Engineering Education, Journal of Engineering Education, 92 (2003) 181-188.
“Engineers will not only ponder problems involving new technologies, but also world cultures, religions, ethics, and economics as well.”
Other quotations from “Engineering education reform: A trilogy” are:
- “If we want to develop Renaissance engineers, multi-talented men and women who will participate in the highest councils, we cannot educate them in vocational schools – even scientifically distinguished vocational schools – which is what many of our engineering colleges are becoming.” S.C. Florman, “Engineering and the Concept of the Elite,” The Bridge, 21 (1991) 11-17.
- “For the most part our faculty are superb “engineering scientists,” but they are not necessarily folks who know a lot about the practice of engineering…. the current faculty are the folks with the largest say in engineering curriculum. Given this, it should not be a big surprise that industry leaders have been increasingly vocal about their discontent with engineering graduates.” W.A. Wulf, “The Urgency of Engineering Education Reform,” The Bridge, 28 (1998) 4-8.
- “First, we’ve reaffirmed that high-quality contributions to engineering education are a valid reason for election to the NAE. This criterion makes it clear that people’s creativity and excellence in engineering education can be rewarded in the same ways as outstanding technological contributions.” W.A. Wulf and G.M.C. Fisher, “A Makeover for Engineering Education,” Issues in Science and Technology, 18 (2002) 35-39.
- “In conclusion I would remind us that with recognition comes responsibility. As NAE members you are the most accomplished and most respected members of the engineering profession. It is up to you to: 1) Widen your horizons. Be a Renaissance engineer – that is, an engineer for the 21st century, 2) Get involved in public policy. Don’t be afraid to run for office. Stand for practical, cooperative solutions. Bring your expertise to the table and make others want to listen to you, 3) Most important, go out and change the world. Make it a better place. Improve the quality of life for all the people of the earth. Isn’t that what engineering is really all about?” George M. C. Fisher speech at the 2001 National Academy of Engineering (NAE) Annual Meeting.
Lorem ipsum dolor sit amet, consectetur adipiscing elit. Ut elit tellus, luctus nec ullamcorper mattis, pulvinar dapibuDr. Peter J. Goodhew provides a free book focusing primarly on the practical aspects of engineering education, Teaching Engineering, All you need to know about engineering education but were afraid to ask. A quotation from the foreword gives an idea about the philosophy behind the book: “I recognise that the vast majority of university teachers want to do a good job for their students but feel that they do not have the time (or sometimes the inclination) to study the literature on engineering education. However they ought to feel that the quality of their teaching should be as high as the quality of their research, and this means being familiar with its background literature. This book is intended to help.” A supplementary book,Teaching Materials Engineering an updated guide, edited by Dr. Goodhew, is also available at UK Centre for Materials Education.
References:
[1] MZ Poh, N. C. Swenson, R. W. Picard, IEEE Transactions on Biomedical Engineering 57 (2010) 1243–1252.
[2] H. D. Critchley, Neuroscientist, 8 (2002) 132–142.s leo.